Virtual
beings boost evolutionary theory
By
Ted Smalley Bowen,
Technology Research News
Anyone plagued by fruit flies can attest
to their speedy proliferation. This trait, while a nuisance in the kitchen,
has long been valued in the lab, where drosophila melanogaster usefully
plays out genetic inheritance at a pace that quickly reveals generational
changes.
Bacteria, whose populations can double in as few as 10 minutes in optimal
environments, are faster still. But to really fast-forward the evolutionary
process, researchers turn to digital organisms living out micro-lifespans
in virtual environments.
Researchers from the California Institute of Technology and Michigan State
University have used computer-generated organisms to confirm a theory
of evolution known as quasi-species theory.
The researchers were aiming to shed more light on the fundamental evolutionary
relationship between mutation and natural selection. Such experiments
could flesh out research in fields like artificial
life and evolutionary
computation.
Mutations are random changes in genetic material. The theory of natural
selection, also known as the survival of the fittest, holds that genes
that are involved with traits that make animals more fit, or more likely
to survive, will tend to increase in a population because the animals
who have them are more likely to pass those genes on to the next generation.
In this model, the key advantage is the speed with which organisms pass
on their genes.
The quasi-species theory refines the strict Darwinian model to predict
that when genetic material is affected by high rates of mutation, a group
of animals with the highest average reproduction rate will be the most
successful in propagating even if that group does not contain the fastest
reproducing individual.
To test the theory, the researchers made populations of self-replicating
digital organisms that mutated and evolved within a portion of their computer
host’s memory, said Claus O. Wilke, a researcher at Caltech. The software
organisms' main function was to replicate themselves, Wilke said. "Each
program contains a little loop that examines itself, and writes a copy
of itself into a new region in memory."
To establish their fitness, the digital organisms performed logical computations
on numbers present in their virtual environment.
Within this digital scheme, an organism’s phenotype, meaning its particular
group of genes, was “mainly the number and type of computations a program
can do,” Wilke said. The pool of genes contained in a group of organisms
is the group's genotype.
The environment distinguished among about 80 different logical computations,
and rewarded organisms’ computational accomplishments by bestowing on
them greater speed. Programs that completed a large number of computations
ran faster, and hence out-competed programs that completed fewer computations,
said Wilke.
The researchers created forty pairs of populations from 40 different ancestors,
and programmed one of each pair to mutate four times faster than the other.
In 12 cases, the dominant genotype that evolved at the lower mutation
rate replicated more than one and a half times faster than its counterpart.
Working with these 12 pairs over 50 generations, the researchers varied
the mutation rates, and found that at higher rates, the genotype with
lower individual fitness rates but higher group rates won out.
Though less prolific, genotypes that were clustered in flatter regions
of a fitness graph - meaning their traits were more redundant - were better
able to withstand the effects of mutation and therefore were more able
to perpetuate themselves than groups with one or several extremely fast
reproducers, but a lower overall average rate, according to Wilke.
“If you have an extremely optimized organism, it is quite likely that
any mutation will seriously impair its replication rate,” Wilke said.
This is because more highly optimized organisms tend to have less redundancy.
For example, in a more finely tuned organism with 100 genes, "all the
genes are different, in order to get the maximum gain out of the 100 genes.
In [a more balanced] organism, there are always several genes that do
the same thing, so that the true number of genes is lower," said Wilke.
"If you hit these genes with mutations, the first organism will feel the
hit immediately, after the first mutation, whereas in the second organism
you can knock out a number of genes before anything serious happens,”
Wilke said. “In other words, under high stress, redundancy is more important
than optimization.”
The experiments showed that a population with a more balanced distribution
of characteristics could out-vie populations with some much fitter individuals
when conditions spur greater mutation. The ability of a population to
withstand mutation could trump another population that had individuals
with as much as a 12-fold replication speed advantage, according to Wilke.
Mutation rates on the order of one per genome per generation, which is
similar to those of some types of viruses, were sufficient to tilt the
evolutionary field away from fast reproducers, he said.
In the real world, high mutation rates are mainly present in RNA viruses,
said Wilke. This is because when a cell divides and duplicates its RNA
or DNA, the duplication often includes
mistakes. RNA and DNA molecules contain long strings of base pairs grouped
into genes, which store information on how to make proteins. These proteins
facilitate many of life's processes.
Most organisms have error correction mechanisms that catch most of those
genetic mistakes. "These viruses have such a high mutation rate... because
they don't have error correction," Wilke said.
Although the study's results were expected, it does advance the use of
digital simulations in understanding evolution, said Lee Spector, an associate
professor of computer science and cognitive science at Hampshire College.
“Studying evolutionary dynamics by experimenting with digital organisms
has enormous potential both for evolutionary theory and for practical
applications," Spector said.
It is also difficult. The complexity of even the simplest biological organism
is well beyond current digital simulations, which in turn are more complex
than analytical models of evolutionary processes, Spector said. “Digital
organisms are abstractions and one must be careful in generalizing the
results of experiments with them."
The study’s methodology involved some key trade-off’s, said Spector. It
used organisms that reproduced asexually, and controlled mutation rates
as an external function, which allowed them to systematically vary mutation
rate, but sacrificed some realism, he said.
Digital organisms are far removed from real-world counterparts, said Jeffrey
Horn, an assistant professor of mathematics and computer science at Northern
Michigan University. "However, they do allow us to exactly control the
environment, which is impossible with any real organisms. This control
allows us to vary any single environmental variable, to fully analyze
the dynamics in every detail [and] to run as many independent trials as
we like." The drawback is that it is difficult to tell if the relationships
the research can isolate and spotlight this way are actually important
in nature, he said.
There's also the possibility that effects in digital populations are not
due to the general dynamics of evolution, but result "from very specific
choices that we have made in the design of our system,” Wilke said.
This can be compensated for by posing questions at a more general level
that do not depend on those microscopic details, said Wilke. The Caltech-Michigan
State researchers did this by looking only at broad categories of replication
rate and robustness, and staying away from detailed conclusions like how
the organisms came to replicate at a certain rate, according to Wilke.
There are some useful conclusions that can be drawn from the research,
said Spector. “The moral... is that robust replicators will sometimes
out-compete more efficient, but less robust replicators, particularly
in disruptive environments,” he said. “So a computer worm that makes only
a few copies of itself, but does so in the context of a wide range of
operating systems and security measures, may spread faster than a worm
that makes hundreds of copies of itself but fails in many environments.”
"The research shows that we must be careful not to turn mutation rates
up too high, or we might evolve robust but suboptimal answers," said Horn.
"Conversely, for artificial life simulations [like] simulated worlds [and]
computer games, we might be encouraged to try very high mutation rights
in the hopes of co-evolving multiple, related, robust species whose evolvability
is more important than their objective fit to the environment," he said.
The researchers next steps include a study of the same evolutionary effect
observed in digitally simulated RNA sequences. They also plan to look
at the evolution of more complex features, according to Wilke. Experiments
that yield significant results might lead to more focused tests on biological
organisms, he said.
While there's no clear-cut commercial application for the digital evolutionary
testbed, this type of experimentation is likely to increase in the next
decade, Wilke said. "I expect a significantly increased number of studies
involving digital organisms over the next five to ten years."
Wilke’s research colleagues were Jia Lan Wang, Charles Ofria, and Christoph
Adami at Caltech, and Richard E. Lenski at Michgan State. They published
the research in the July 19, 2001 issue of the journal Nature. The research
was funded by the National Science Foundation (NSF) and the National Aeronautics
and Space Agency (NASA).
Timeline: 5-10 years
Funding: Government
TRN Categories: Artificial Life and Evolutionary Computing
Story Type: News
Related Elements: Technical paper, “Evolution of Digital
Organisms at High Mutation Rates Leads to Survival of the Flattest” Nature,
July 19, 2001.
Advertisements:
|
October
10, 2001
Page
One
Tiny tubes make logic
circuits
Mobile radios make intranet
Quantum code splits secrets
Computer tells convincing
story
Virtual beings
boost evolutionary theory
News:
Research News Roundup
Research Watch blog
Features:
View from the High Ground Q&A
How It Works
RSS Feeds:
News | Blog
| Books 
Ad links:
Buy an ad link
Advertisements:
|
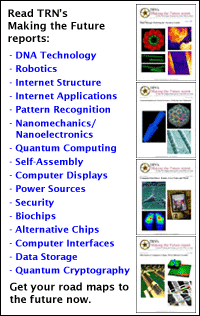
|
|
|