X-rays
light 3-D microscope
By
Kimberly Patch,
Technology Research News
Researchers in the burgeoning fields of
materials science and nanotechnology need to know exactly how tiny objects
arrange themselves in three-dimensional space. Pinning down the precise
locations of individual grains of next-generation materials and minuscule
pieces of microscopic machines can go a long way toward making them more
useful.
A group of researchers from Oak Ridge National Laboratories has taken
the field of microscopy a significant step forward with a three-dimensional
x-ray technique that shows attributes like molecular density and chemical
distribution within crystalline materials like metal and ceramics.
The technique allows scientists to resolve, or bring into focus three-dimensional
features as small as a few tenths of a micron, which is smaller than a
single bacterium. A micron is one thousandth of a millimeter.
The method would allow engineers to look more deeply within the materials
that form airplane wings to gain a better understanding of how metal fatigue
progresses, and to examine how silicon atoms grow into the wafers used
to make computer chips in order to their manufacture.
There are several types of microscopes. Optical microscopes bounce visible
light off objects, showing surface information like spacing and color.
They can resolve features as small as 0.2 microns, or about half the size
of the wavelengths of visible light, which range from 0.4 to 0.7 microns.
Transmission x-ray microscopes take advantage of the smaller wavelengths
of x-rays, which range from 0.001 to 0.00001 microns, and x-rays' ability
to penetrate materials, which allows them to be used to measure things
like bone density.
Electron microscopes use beams of electrons to image much smaller objects.
Electron wavelengths as small as 0.000004 microns can resolve features
as small as 0.00002 microns, which is nearly as small as an individual
atom. Because electrons cannot penetrate very far into substances, however,
the range of electron microscopes is limited to surfaces, or in the case
of transmission electron microscopes, a depth of only a few hundred atoms.
Although x-rays penetrate more deeply into materials, it has been historically
difficult to use them to image extremely small features in three dimensions
because varying penetration rates have to be accounted for and because
x-ray diffraction shows crystal structure and strain rather than the actual
positions of objects.
The Oak Ridge scientists solved these problems mathematically.
Crystals are made up of atoms or molecules stacked into regular, periodic
structures, or lattices. X-ray pictures of crystals are actually pictures
of the way x-rays are affected when they diffract, or change direction,
while traveling through the crystal lattice.
The x-ray scattering is recorded as a collection of spots because x-rays
reflect off the atomic planes of crystals like light reflecting off a
series of mirrors, said Bennett Larson, group leader for x-ray diffraction
at Oak Ridge National Laboratory. "Each part of the crystal grain in the
sample has about 20 to 50 reflections, or spots, and we need to catch
10 or more to get good results," he said.
These patterns of light and dark spots can be mathematically analyzed
to reveal the crystal structure. "The x-ray diffraction images [are] not
correct spatial images of the material in the sense of, say, medical x-rays,
but... diffraction patterns... that can be computer-analyzed to determine
the structure, orientation and strain of the material that would produce
such a pattern," said Larson.
To extract information about the three-dimensional structure of the crystal
from these patterns, the researchers found a way to use triangulation.
"The x-rays reflect at large angles from a narrow... microbeam of x-rays,"
said Larson. "We know where the x-rays entered the sample but we do not
know where along the beam" the reflected x-rays come from, he said.
The researchers knew the location of one point in an x-ray trajectory
from the resulting spot in the pattern. By periodically obstructing parts
of the reflected beam and recording the point of obstruction they found
a second point. Once they knew the location of these two points, they
extended the line between them down to the original x-ray beam. The portion
of the sample that was diffracting the x-rays lay at the intersection
of the line and the beam.
The method is "somewhat like trying to determine how deep in the batters
box a batter was standing when a ball was hit into left field," said Larson.
"We cannot determine this solely from where the fielder catches the ball,
but if we also know that the ball went directly over the shortstops head,
for instance, we could draw a straight line from the fielder to the shortstop
and extend the line to the batters box. This would tell accurately where
the batter must have been standing," he said.
A differential-aperture, or knife-edge device, which focuses diffraction
pattern depth, was the critical missing piece for three-dimensional x-rays,
said Larson. "Previously, the superimposed x-ray scattering patterns...
along the [x-ray] microbeam could not be sorted out as a function of depth,"
he said.
The researchers' differential-aperture x-ray microscopy (DAXM) technique
uses a platinum wire to record the x-ray diffraction. The way the physics
works out presented a problem, however. For the wire to properly absorb
the x-rays it needed to be at least 50 microns thick, and yet have only
a one-micron wide aperture, or opening. This made it "a very narrow tunnel
that will [record] only about one or two degrees of divergence angle,
[missing] almost all of the scattering," said Larson.
To get around the problem, the researchers made a virtual aperture by
moving a solid wire in one-micron steps to take a series of overlapping
pictures. They used existing computer analysis programs to digitally subtract
each diffraction pattern from the previous one, yielding consecutive one-micron-wide
slices.
The researchers tested the method by measuring the orientation and strain
gradients in a bent silicon wafer, which they could check using calculations.
They also measured the rotational distortions produced when materials
are deformed, and used the method to image the crystalline structures
of aluminum and copper.
The researchers have found unexpected results. "We were surprised at the
high degree of crystal perfection within the grains of the... aluminum,"
Larson said. They also found that the way parts of the copper lattice
were rotated was more complicated than expected. The samples had "a much
richer structure than expected," he said.
The potential impact of the work is "enormous," said Yong Chu, a staff
scientist at Argonne National Laboratory. "This is the first work unequivocally
demonstrating that structural change in solid can be resolved better than
submicrometer resolution in all three dimensions."
This makes it feasible to measure crystal structures of materials in three
dimensions without destroying the specimen, said Chu. Most engineering
materials, from metal to ceramics, are crystalline. "It opens up a wide
range of interesting applications. For example, one can now study the
fracture behavior of materials with submicron spatial resolution so that
the theory of fracture mechanics can be refined. Much safer airplanes
could be produced," he said.
The technique could also be used to study material strain in microelectronics
to improve failure prediction models and produce better and cheaper chips,
said Chu.
The technique has a couple of minor drawbacks, Chu added. It cannot be
used for materials that are sensitive to radiation damage. Biological
crystals and some polymers may be damaged during the process because the
technique uses white x-rays, which span a wider energy bandwidth and thus
cause far more photons to strike a sample than regular monochromatic x-rays.
The technique also requires facilities that can produce both regular monochromatic
and white x-rays, which are not common, Chu said. "There is a considerable
amount of investment associated with adopting the... technique," he said.
The Oak Ridge researchers are now using the technique to look at the underlying
physics of growing, heating, deforming and fracturing a wide range of
materials including crystals and composite materials, said Larson.
Larson's research colleagues were Wenge Yang, Gene E. Ice, John D. Budai
and Jon Z. Tischler. They published the research in the February 21, 2002
issue of Nature. The research was funded by the Department of Energy (DOE).
Timeline: Now
Funding: Government
TRN Categories: Data Acquisition; Data Representation and
Simulation
Story Type: News
Related Elements: Technical paper, "Three-dimensional X-ray
Structural Microscopy with Submicrometer Resolution," Nature, February
21, 2002.
Advertisements:
|
February
27, 2002
Page
One
Handheld DNA detector near
Microscopic antenna
unzips DNA
Cheaper LED shines
the right light
X-rays light 3-D microscope
Nanotubes pack power
News:
Research News Roundup
Research Watch blog
Features:
View from the High Ground Q&A
How It Works
RSS Feeds:
News | Blog
| Books 
Ad links:
Buy an ad link
Advertisements:
|
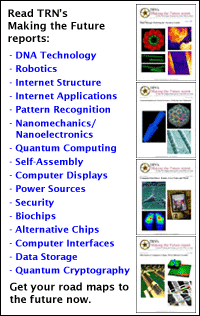
|
|
|