Silicon
chips set to go atomic
By
Eric Smalley,
Technology Research News
Prototype quantum computers, which use
atoms or subatomic particles to carry out computations, are laboratory
oddities that run gamut from lasers fired into special crystals to test
tubes of liquid placed in the same type of machine that produces medical
MRIs.
Quantum computers could be fantastically fast at solving large problems
like cracking secret codes, but they remain largely theoretical, which
is why researchers are trying different approaches to see if it's possible
to make large, practical computers that use the states of subatomic particles
to store and process information.
Researchers from Stanford University and Keio University in Japan are
exploring familiar ground in an attempt to make quantum devices as similar
as possible to ordinary, classical computers. The team has come up with
a way to build quantum computers entirely out of the traditional material
of computers -- silicon.
"Silicon-based architectures are exciting because of all the engineering
that has already gone into silicon technology over the last 40-plus years,"
said Thaddeus Ladd, a researcher at Stanford University.
Quantum computers use atoms or subatomic particles -- qubits -- to represent
bits of information. The nucleus of an atom can act like a tiny magnet,
and depending on which way its magnetic field is oriented, it represents
a 1 or a 0. Conventional computers use the presence or absence of electric
current running through transistors to indicate the 1s and 0s of digital
information.
When an atom is isolated from its environment the nucleus is in the weird
quantum state of superposition, meaning it is in some mixture of all possible
states. A qubit in superposition is a mix of 1 and 0, and a string of
qubits in superposition can represent every combination of 1s and 0s at
the same time.
The power of a quantum computer comes from this ability to represent and
check every combination at once to find the number that represents the
solution to a problem. Ordinary computers have to check each possible
answer one at a time. "One [quantum] computer does the work of many computers,"
said Ladd.
In the largest prototype quantum computer to date, researchers used liquid
nuclear magnetic resonance (NMR) technology to manipulate seven qubits.
NMR, which is the technology behind magnetic resonance imaging (MRI) machines,
uses magnetic fields and radio waves to shift and measure the atomic nuclei
in the molecules that make up a liquid.
Researchers generally agree that liquid NMR quantum computers, however,
cannot be made larger than 10 qubits because the strength of the radio
signals from the qubits decreases exponentially compared to the noise
with each additional qubit. To live up to its vast potential, a quantum
computer must contain thousands or millions of qubits. Today's classical
computers contain millions of transistors.
The Stanford and Keio University researchers' proposal also uses NMR technology,
but in a solid form. Their idea is to embed atoms of the silicon isotope
silicon29, whose nuclei act like magnets, in ordinary silicon, whose nuclei
do not. This isolates the silicon29 nuclei, reducing the noise problem.
Another challenge to making quantum computers is preserving the fragile
states of qubits. Energy from the environment can disturb the qubits,
causing them to decohere. Decoherence is like when a spinning top falls
over or is touched so that it changes direction, said Ladd. The longer
a qubit remains coherent, the more operations a quantum computer can carry
out.
The embedded silicon scheme addresses the decoherence problem as well,
protecting the magnetic nuclei so that their quantum states last longer.
The researchers' scheme calls for using a string of silicon29 nuclei to
form a qubit. If ordinary silicon wafers were cut so the crystal structure
of the edges forms steps, silicon29 atoms deposited on the edges would
move to the corners of the steps, making chains, said Ladd.
Measuring the magnetic orientation of a chain of a few hundred atomic
nuclei is difficult compared to measuring the magnetization of a group
of billions of nuclei in the molecules of a liquid. "Such a small nuclear
magnetization has never before been measured," said Ladd.
The researchers' architecture calls for measuring and controlling the
qubit chains by putting them in magnetic fields of different strengths
so that each chain responds to a different radio frequency. "Essentially
each qubit has its own radio channel," said Ladd. "By adjusting the frequency
of the radio waves we generate with an antenna, we can tune in to each
nucleus. These radio frequency signals can rotate the nuclei any way we
want," he said.
The quantum computer would carry out logic operations through intricate
sequences of rotations.
The scheme is not the first proposed for making a quantum computer out
of silicon. University of Maryland researcher Bruce Kane devised a plan
to make qubits out of phosphorous atoms placed at regular intervals in
a silicon chip. The qubits in that scheme would be more prone to decoherence
and would likely be even more challenging to read than those in the researchers'
all-silicon approach, said Ladd.
Kane's proposal may have more promise in a long run, however, because
it is more likely to lead to machines with the thousands or millions of
qubits necessary to make practical quantum computers, said Ladd. "The
big difference is that our proposal is more short-term and realistic,
while Kane's is more far-reaching and therefore more uncertain," he said.
The Stanford and Keio University researchers' proposal is interesting
and would probably be easier to implement than other types of silicon
quantum computers, but it will not scale up to large numbers of qubits,
said Kane.
The researchers' work may solve some of the problems in current NMR computers,
said Eli Yablonvitch, a professor of electrical engineering at UCLA. Using
strings of silicon29 nuclei would have many advantages over using liquids,
he said.
However, "it would remain painfully slow, with a clock speed of less than
1 kHz," Yablonvitch said. Magnetic fields cause atomic nuclei to resonate
at relatively low frequencies. The radio signals used to control the qubits
must match their low resonant frequencies, which limits the speed at which
the computer can carry out instructions.
For this reason, other research teams are working with electrons rather
than atomic nuclei. This type of silicon quantum computer "would have
many of the same advantages but could have a clock speed of 1 GHz," said
Yablonvitch. Like nuclei, electrons also act like tiny magnets, but they
can be manipulated with ultrafast pulses of laser light. The downside
to using electrons is that their quantum states are short-lived compared
to the states of nuclei.
It's difficult to estimate how long it will take to develop full-scale
quantum computers, said Ladd. It is likely to take at least 20 years,
but this is "wild speculation," he said.
Ladd's research colleagues were Jonathan Goldman, Fumiko Yamaguchi and
Yoshihisa Yamamoto of Stanford University and Eisuke Abe and Kohei Itoh
of Keio University in Japan. They published the research in the July 1,
2002 issue of the journal Physical Review Letters. The research was funded
by the Japan Science and Technology Agency, NTT and the Defense Advanced
Research Projects Agency (DARPA).
Timeline: > 20 years
Funding: Government, Corporate
TRN Categories: Physics; Quantum Computing and Communications
Story Type: News
Related Elements: Technical paper, "All-Silicon Quantum
Computer," Physical Review Letters, July 1, 2002
Advertisements:
|
July
24/31, 2002
Page
One
Disks set to go ballistic
Two-step
queries bridge search and speech
Implant links
nerve cells to electronics
Silicon chips set to
go atomic
Light switch
promises powerful computers
News:
Research News Roundup
Research Watch blog
Features:
View from the High Ground Q&A
How It Works
RSS Feeds:
News | Blog
| Books 
Ad links:
Buy an ad link
Advertisements:
|
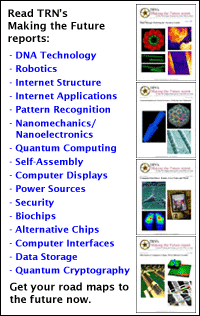
|
|
|