DNA
prefers diamond
By
Kimberly Patch,
Technology Research News
Scientists have shown that DNA is an extremely
useful molecule -- it can sense other substances and can automatically
arrange itself into microscopic structures.
Tapping the talents of the largest known molecule to sense pathogens like
those used in bioterrorism, however, means solving a couple of problems.
In order to operate in the field, the delicate molecule needs a stable
portable environment and a connection to electronics that will reveal
immediately what the DNA has sensed.
Both challenges would be solved by physically and electronically attaching
biological molecules to the silicon wafers computer chips are made from,
but that has proved difficult.
A research team from the University of Wisconsin at Madison, Argonne National
Laboratory and the Naval Research Laboratory has found that DNA prefers
diamond.
What's more, there's electricity involved. The researchers found that
when DNA is attached to diamond, it is possible to electronically detect
the changes in DNA that mark its connection with another molecule.
The combination of a very stable diamond-DNA interface and direct electronic
readout could lead to real-time biosensors, including devices that continuously
monitor for pathogens, said Robert Hamers, a chemistry professor at the
University of Wisconsin at Madison.
Diamond wasn't the obvious choice. The researchers first worked out, with
limited success, chemistry for attaching DNA to silicon. "We always found
that there was some slow degradation of the interfaces that we reasoned
was intrinsic to the chemistry of silicon," said Hamers.
Today's gene chip technologies already commonly attach biological molecules
to silicon dioxide, or glass, a method that works well in the lab, but
is less appropriate for the field. "Glass is slightly soluble in water,
and so when exposed to water for some time, the outermost layer of glass
and the biological... layer that it is attached to simply fall off into
solution," Hamers said.
Glass works well for biomedical applications because they tend to use
molecules just once, said Hamers. "However, our target application is
more along the lines of real-time, continuous monitoring," he said.
Gold is another common surface for attaching biological molecules, but
"the molecules have sulfur atoms on the end that bond weakly to the gold
surface. This makes nice layers, but again they're not stable for long
periods of time," Hamers said.
Biological, or organic, molecules contain carbon, which is the sole atomic
ingredient of diamond. "Since carbon-carbon bonds are stronger than silicon-carbon
or silicon-silicon bonds we reasoned that diamond surfaces would be more
stable," said Hamers. "We decided to see if the chemistry we developed
for modifying silicon with DNA would work with diamond," he said. With
some modifications, it worked.
The research group developed a method to chemically modify the diamond
surface with organic chemical groups that served as good attachment points
for biomolecules, said Hamers. "Once that was in place, the rest was reasonably
straightforward," he said.
The researchers used ultraviolet light to chemically bond amine molecules
to the diamond. Amine is similar to ammonia, and is a product of decomposing
biological material. The researchers used a layer of cross-linker molecules
to attach DNA to the amine layer.
The carbon-carbon bonds between biological molecules and diamond are extremely
strong and are not subject to the degradation processes that plague other
materials, said Hamers. The key was "simply recognizing the carbon-carbon
bonds are very strong and very stable, and that if we could link biological
materials to something like diamond, it would probably be incredibly stable,"
he said.
Diamond has a couple of other advantages for real-time field applications
like biological sensors, said Hamers. Diamond, like silicon, is a semiconductor,
meaning it conducts electricity in a way that can be controlled to make
useful electronics. Diamond can also be grown in extremely thin layers,
and can be easily integrated with silicon, said Hamers. "You could make
an integrated microelectronic device with silicon and diamond," said Hamers.
The drawback to using diamond is that it takes longer to process than
silicon, glass or gold; researchers have made recent progress, however,
in efficiently producing very thin diamond films. The diamond surface
the researchers used to attach DNA was 500 nanometers thick, which is
150 times thinner than a human hair. A nanometer is one millionth of a
millimeter.
Just as important as the physical connection is the electronic one. "Using
diamond, we have recently been able to sense DNA hybridization and protein
binding entirely using electronic means," said Hamers. "We can make the
diamond surface part of an electrical circuit, and when DNA binds to it
we can sense that electrically."
The common alternative is optical sensing, which uses light to measure
changes in DNA. Optical sensing is expensive and requires a lot of power,
said Hamers.
In contrast, electrical sensing is inexpensive, requires little power,
and integrates well with existing electronics, Hamers said. "By going
to electronic detection, one has the possibility of leveraging all the
power of microelectronics -- high degrees of parallelization, integration,
small size, on-board signal amplification and processing, and [even] build-in
communications," he said.
The method is ready now to apply to practical devices, said Hamers.
The researchers are working to make miniaturized versions of the system
with all the electronics on the same chip, said Hamers. This would provide
"a complete biosensor system on a chip [including] the sensor itself [and]
all the fluid handling and signal processing," components, he said.
Eventually, the method could be used for a sort of bio-cell phone, Hamers
said. Such a device could be "carried around or placed in high-traffic
areas to continuously monitor for chemical or biological pathogens," he
said. Because the sensing DNA can be read electronically, when the device
encountered a pathogen it could immediately generate a warning signal,
he said.
Because the diamond surfaces appear to be very stable they could be very
useful, said Robert Corn, professor of chemistry at the University of
Wisconsin at Madison.
Corn has teamed up with Hamers in a project to use the method to make
better DNA arrays for biological and computing applications. "The robust
surface chemistry will allow us to perform many enzymatic... reactions
on the DNA monolayers without degradation," said Corn. "The electronic
sensing abilities of DNA hybridization of diamond are also potentially
very useful for the sensing of DNA in both [biological and computing]
applications," he said.
Hamers' research colleagues were Wensha Yang, Wei Cai, Tanya Knickerbocker,
Tami Lassiter and Lloyd M. Smith of the University of Wisconsin at Madison,
Orlando Auciello, John A. Carlisle, Jennifer Gerbi and Dieter M. Gruen
of Argonne National Laboratory, and James E. Butler and John N. Russell,
Jr. of the Naval Research Laboratory.
They published the research in the November 24, 2002 issue of Nature Materials.
The research was funded by the Office of Naval Research (ONR), the Wisconsin
Alumni Research Foundation (WARF), and the National Science Foundation
(NSF).
Timeline: < 1 year
Funding: Government; University
TRN Categories: Biology; Biotechnology;Materials Science
and Engineering; Nanotechnology
Story Type: News
Related Elements: Technical paper, "DNA-Modified Nanocrystalline
Diamond Thin-Films As Stable, Biologically Active Substrates," Nature
Materials, November 24, 2002.
Advertisements:
|
December
11-25, 2002
Page
One
DNA prefers diamond
Material soaks up the sun
Design links quantum bits
Microscopic mix
strengthens magnet
Laser pulses could
speed memory
News:
Research News Roundup
Research Watch blog
Features:
View from the High Ground Q&A
How It Works
RSS Feeds:
News | Blog
| Books 
Ad links:
Buy an ad link
Advertisements:
|
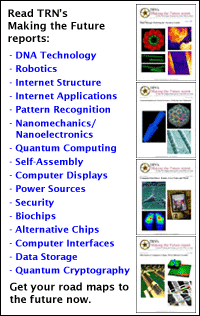
|
|
|