Material grabs more sun
By
Kimberly Patch,
Technology Research News
One way to make solar cells more efficient
is to find a material that will capture energy from a large portion of
the spectrum of sunlight -- from infrared to visible light to ultraviolet.
Energy transfers from photons to a photovoltaic material when
the material absorbs lightwaves that contain the same amount of energy
as its bandgap. A bandgap is the energy required to push an electron from
a material's valence band to the conduction band where electrons are free
to flow.
The trouble is, most photovoltaic materials absorb a relatively
narrow range of light energy. The most efficient silicon solar cells capture
about 25 percent of the sun's energy. Multijunction solar cells combine
several materials to capture multiple bands of photonic energy. Today's
most efficient combination -- germanium, gallium arsenide and gallium
indium phosphide -- boosts efficiency to 36 percent, but is relatively
difficult to make and therefore expensive.
Researchers from Lawrence Berkeley National Laboratory, the University
of California, and the Massachusetts Institute of Technology have engineered
a single material that contains three bandgaps. The material is capable
of capturing more than 50 percent of the sun's energy, said Wladek Walukiewicz,
a senior staff scientist at the Lawrence Berkeley National Laboratory.
The material could lead to relatively inexpensive, highly-efficient
solar cells. Such cells would be much simpler than today's high-end multijunction
solar cells because the three bandgaps reside in a single material, said
Walukiewicz.
The researchers have manufactured a prototype single-junction
3-band semiconductor from the material. Although the concept of a multiband
material was proposed in 1960, the researchers' prototype is "we believe,
the first realization of a multiband semiconductor," said Walukiewicz.
The researchers were working on making a three-junction photovoltaic
cell when they accidentally made a material that had a split bandgap.
Once they realized the nature of the material, they reverse-engineered
their inadvertent discovery to figure out how it happened.
The key turned out to be replacing some of the atoms of a material
that is strongly electronegative with atoms of a material that is even
more electronegative to create a highly mismatched alloy. The introduced
atoms split the conduction band.
The researchers found that in the case of zinc-manganese-tellurium,
instead of splitting the conduction band, introduced oxygen molecules
"formed their own band well separated from the original conduction band,"
said Walukiewicz. "As a result we had a semiconductor with three bands
-- the valence band and two conduction bands.
The difference between the material's valence band and first conduction
band, or the amount of energy needed to push an electron from one to the
other, provided a bandgap that absorbs photons that contain 1.8 electron
volt. The difference between the two splits made for a 0.7 electron volt
bandgap. And the difference between the valence band and second conduction
band made for a bandgap of 2.6 electron volts. These three gaps cover
much of the solar spectrum. An electron volt is the work required to move
an electron through a potential difference of one volt.
The researchers made the material by forcing oxygen into zinc-manganese-tellurium.
They did so by heating a mix of zinc-manganese-tellurium and oxygen with
a laser so that it melted and then recrystallized. The laser made this
happen fast enough that oxygen could not escape, and so was forced to
become part of the crystal, said Walukiewicz.
The researchers are working on making a practical solar cell from
the material, according to Walukiewicz. This requires forming p-type and
n-type versions of the material. The valence band of p-type, or positive-type
material has missing electrons, or holes. The conduction band of n-type,
or negative material, contains electrons. A junction between the two types
of materials creates a place where, when photons are absorbed, electrons
move to the p-type material and holes toward the n-type to create an electrical
current.
It will take to three years to assess the technical feasibility
of the multiband solar cell, according to Walukiewicz.
Walukiewicz's research colleagues were Kin Man Yu, J. Wu, W. Shan,
and J. W. Beeman from Lawrence Berkeley National Laboratory, M. A. Scarpulla,
and O. D. Dubon From Lawrence Berkeley National Laboratory and the University
of California, and P. Becla from the Massachusetts Institute of Technology.
The work appeared in the December 12, 2003 issue of Physical Review
Letters. The research was funded by the Department of Energy (DOE).
Timeline: 2-3 years, Unknown
Funding: Government
TRN Categories: Materials Science and Engineering; Energy
Story Type: News
Related Elements: Technical paper, "Diluted II-VI Oxide
Semiconductors with Multiple Band Gaps," Physical Review Letters, December
12, 2003
Advertisements:
|
April 21/28, 2004
Page
One
Material grabs more sun
Spoke polarization
tightens focus
Molecule makes electric
motor
Optical quantum memory
designed
Briefs:
Printer writes
micro 3D objects
Tiny rotors spin
into place
Nanotube forms drive
shaft
Photons teleported
six kilometers
Magnets align
nanotubes in resin
Sturdy quantum
crypto proposed
News:
Research News Roundup
Research Watch blog
Features:
View from the High Ground Q&A
How It Works
RSS Feeds:
News | Blog
| Books 
Ad links:
Buy an ad link
Advertisements:
|
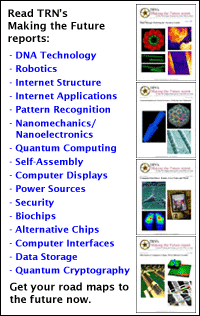
|
|
|