Scheme reverses light pulses
By
Eric Smalley,
Technology Research News
Getting
out exactly what you put in is easier said than done, especially for something
as ephemeral as a light pulse. Bouncing and redirecting light pulses with
mirrors and lenses inevitably distorts them and diminishes their energy.
Researchers at Stanford University have developed a method for accurately
time-reversing electromagnetic pulses, making it possible to receive a light
pulse and return a replica of exactly the same size, shape and wavelength.
"The outgoing pulse is a perfect time-reversed replica of the original pulse,
without any distortion," said Mehmet Fatih Yanik, a researcher at Stanford.
The time reversal scheme could be used to boost optical and microwave
communications, said Yanik. "The time-reversal process can be used to compensate
for dispersion and to increase the bandwidth of communications systems,"
he said. "In the microwave domain, it could significantly improve radar
systems and signal processing."
The theoretical technique could also lead to room-temperature, on-chip
quantum photonics and quantum computing devices, said Yanik. Quantum photonics
involves controlling individual photons for communications applications
like quantum cryptography. Optical quantum computing involves using properties
of individual photons to carry out computations.
The time-reversal method is an extension of the researchers' earlier
technique for briefly stopping light pulses using photonic crystal. Photonic
crystal is material that blocks or channels specific wavelengths of light
using refraction, or the bending of light, at the boundaries of tiny, regularly
spaced holes or rods in the material. In the researchers' light-stopping
and time-reversing systems, the photonic crystal contains tiny cavities
that vibrate at the same frequencies as the light pulses.
The equipment needed is relatively simple, said Yanik. Conventional
time reversal schemes require high-power lasers and very special materials,
he said. "All we need is tunable resonators, which can be [fabricated] with
conventional microprocessing technologies."
The time-reversal photonic crystal system contains two sets of cavities,
or resonators. One receives an incoming pulse and the other generates an
outgoing pulse. "The light pulse first enters the photonic crystal, which
consists of many coupled resonators," said Yanick. "As the photon pulse
propagates inside the crystal, we shift the relative resonance frequencies
of the cavities to transfer photons from one subsystem of cavities to another,"
he said.
The second set of cavities is tuned to exactly invert the pulse
and send it in the direction the original pulse came from, said Yanik.
Key to the researchers' system is the ability to tune the resonant
frequencies of the cavities on-the-fly, which in turn changes the frequency
of the trapped light. The challenge was finding a way to change the resonant
frequencies very quickly -- as many as 100 billion times the second -- without
changing the shapes of the pulses. Light pulses are made up of multiple
wavelengths, and pulses tend to spread out when they travel through matter
because wavelengths are altered to different degrees. Careful timing of
the resonant frequency modulation assures that each wavelength is altered
in proportion to the others in order to preserve the pulses.
One hundred cavities is sufficient to time-reverse a 20-gigahertz-wide
pulse centered at 200 terahertz, according to Yanik. The terahertz portion
of electromagnetic spectrum is below visible light, between microwaves and
infrared light.
The researchers are close to building a microwave prototype of their
original light-stopping system, said Yanick. Making the light-stopping and
time-reversal techniques practical will require high-quality microcavities
that can change frequencies without losing much of the light's energy, he
said. "Although it might take a while, these challenges are not fundamental
and can be overcome with proper engineering."
Microwave applications could be practical in one to three years;
optical applications could be practical in five to ten years, said Yanick.
Yanik's research colleague was Shanhui Fan. The work appeared in
the October 22, 2004 issue of Physical Review Letters. The research
was funded by Stanford University.
Timeline: 1-3 years, 5-10 years
Funding: University
TRN Categories: Optical Computing, Optoelectronics and Photonics
Story Type: News
Related Elements: Technical paper, "Time Reversal of Light
with Linear Optics and Modulators," Physical Review Letters, October 22,
2004
Advertisements:
|
April 6/13, 2005
Page
One
Stories:
Programmed DNA forms
fractal
Dialogue system juggles
topics
Scheme reverses light
pulses
View from the High Ground:
Joan Feigenbaum
Briefs:
Tough material
gets functional
Water shifts rubber's
shape
Interference
scheme sharpens focus
System forms light
necklace
Trapped light
pulses interact
Optics demo does
quantum logic
Strained
material cleans up memory
News:
Research News Roundup
Research Watch blog
Features:
View from the High Ground Q&A
How It Works
RSS Feeds:
News | Blog
| Books 
Ad links:
Buy an ad link
Advertisements:
|
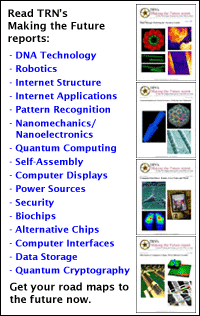
|
|
|