Biology
harbors hidden complexity
By
Kimberly Patch,
Technology Research News
Complicated systems are all around us.
It takes hundreds of networked computers to keep a Boeing 777 aloft and
thousands of interrelated genes to form and maintain a living being. Most
of the time, however, the systems don't look so complicated.
This is because the complexity is hidden in the networks of interactions
carried out by the basic components that make up the systems.
Researchers from the California Institute of Technology and the University
of Michigan who are attempting to unravel the mathematical rules of network
complexity have shown that there are similarities in this mid-level networking
behavior of engineered and natural systems.
"I was struck by how much the engineering-level design [of biological
systems] looks like engineering, despite the fact that the components
were completely different and the system-level behavior might be very
different," said John Doyle, a professor of control and dynamical systems,
bioengineering, and electrical engineering at the California Institute
of Technology.
Finding the mathematics behind the complexity could help unravel biological
mysteries and maintain the world's increasingly interrelated networks.
This type of complexity in biological systems is often hidden in idealized
laboratory settings, said Doyle. This is because what biology gains from
the complexity is robustness, or an ability to deal with fluctuations
in the environment that aren't present in the lab. The same is true for
an airplane in a simple wind tunnel experiment, he said.
Both artificial and biological systems have high-level behavior that is
relatively straightforward. In a network, for example, the behavior might
be transferring a file, and in the human body maintaining a certain temperature.
And at the lowest level, both types of systems are made of just a few
basic building blocks. All of computing is based on a small set of basic
logic operations. Similarly, most organisms use the same codons, which
are sets of three bases that make up the logical units of DNA. Codons
specify the amino acids needed to build the many types of proteins that
work together to carry out life's functions.
"The simplicity is at the high-level behavior and at the lowest levels,
but the layers in between are just not simple. That doesn't mean they
don't obey principles," said Doyle.
The researchers found that the same principles cover both artificial and
biological systems. "The organizational... principles that govern the
layers of protocols, modules and feedback that lie in between are far
more alike than is commonly realized," said Doyle.
The evolution of the Internet, for instance, shows parallels to biological
evolution "after you see how to look at things the right way," said Doyle.
Protocols, the software programs that lay out the rules and regulations
of the network, are the hidden layer of complexity that keeps the network
running relatively smoothly, he said.
In addition to this common structure of simple building blocks, simple
results and mid-level complexity, artificial and biological networks both
show robustness, or an ability to adapt, according to Doyle.
Robustness is what allows biological organisms to, for instance, grow
in almost every environment on earth, including hydrothermal vents 2,600
meters under the ocean, where temperatures are near boiling, the pressure
is 3,821 pounds per square inch and the single-cell microbe Methanococcus
jannaschii thrives. This robustness is manifest in the number of genes,
or sequences of codons, organisms have. Even though less than 300 genes
are essential to an E. coli bacteria, it contains about 4,000, said Doyle.
Along with robustness, however, the complexity brings a type of fragility,
Doyle said. Although large, multicellular organisms are unaffected by
the death of individual cells, certain malfunctions in the middle-layer
control systems of one or a few cells can balloon into fatal autoimmune
diseases or cancer, he said. These failures are due to a cascade effect
among the complicated connections of that layer.
Complicated mechanical systems are also prone to extreme fragility, said
Doyle. A small change in an otherwise intact control system could cause
wild, catastrophic behavior. A relatively simple software bug, for example,
could turn a collision-avoidance system into a collision-seeking system,
according to Doyle.
The same is true of the network of networks that make up biological ecosystems,
said Doyle. Epidemics, starvation, forest fires, and takeovers by exotic
species typically involve small initiating perturbations that cascade
into network and system-wide events, he said.
Cascading failures are relatively rare in nature, however, because systems
that have them frequently die off, said Doyle. "If they were not rare,
we would not exist," he said.
Artificial networks of networks are also prone fragility, said Doyle.
The perpetrators of the September 11 disaster were able to cause so much
damage because they tapped into networks to cause cascading failures,
said Doyle. The hijackers used one complicated system -- an airplane --
to attack another complicated system -- a building. The effect went even
further because the World Trade Center towers were key components of yet
another network, the global financial markets, said Doyle. "Only by using
networks against themselves can such large events occur," he said.
The researchers are currently refining the theory by exploring similarities
in the mathematics of real-world problems that may not at first appear
related. "The math underlying our theories of the Internet and biology
may have deep similarities, but that won't necessarily be apparent" to
experts in those individual areas, Doyle said.
The concepts already apply to the control software of complicated systems
like military and commercial aircraft, manufacturing process control and
smart weapons, said Doyle. "Modern aircraft and automobiles are designed
with billion-dollar computer-aided design systems, and are maintained
with vast networks of diagnostic software. This is not just any old software,
but depends on lots of deep engineering understanding of how the networks,
and not just the components of the systems, work," said Doyle.
They can also be applied, however, to unravel the secrets of biology by
reverse-engineering complex biological systems, and to improve many other
artificial networks, said Doyle.
The emphasis in biology is now shifting from identifying individual components
and molecules to the study of the vast networks that biological molecules
create, which regulate and control life, he said. "We shouldn't expect
biology and medicine to be simpler than [engineered systems], and we will
need similar systems-level theory and software," he said.
In addition, our daily lives are increasingly dominated by our interactions
with a wide variety of networks, including, energy, health, financial,
political, and data networks, which in turn interact with the local and
global environments, said Doyle.
As these networks are increasingly integrated there is both unprecedented
promise and unprecedented risk, he said.
Currently, "a lightning strike in one state can cause a power outage in
another state. A hacker on another continent can deny Web access. A single
firm can trigger a global financial crisis. A software bug can cause a
rocket, airplane, or automobile to crash."
These catastrophic events still have limited impact because the different
types of networks are fairly isolated from each other, said Doyle. As
the world gets more interconnected, however, "this is changing," he said.
Eventually, "everything will boil down to how well we can understand the
complexity of our various networks and their interactions."
Doyle's research colleague was Marie E. Csete of the University of Michigan.
They published the research in the March 1, 2002 issue of the journal
Science. The research was funded by the Department of Defense (DOD), the
National Science Foundation (NSF) and the National Institutes of Health
(NIH).
Timeline: Now
Funding: Government
TRN Categories: Biology; Engineering; Theory
Story Type: News
Related Elements: Technical paper, "Reverse Engineering
of Biological Complexity," Science, March 1, 2002.
Advertisements:
|
March
6, 2002
Page
One
Interactive robot
has character
Atomic cascade broadens
laser
Biology harbors
hidden complexity
Heat engines
gain quantum afterburner
Nanotubes branch out
News:
Research News Roundup
Research Watch blog
Features:
View from the High Ground Q&A
How It Works
RSS Feeds:
News | Blog
| Books 
Ad links:
Buy an ad link
Advertisements:
|
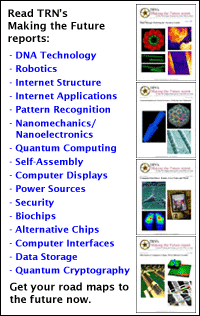
|
|
|