Magnets
channel biomatter
By
Chhavi Sachdev,
Technology Research News
Fluid is useful for transporting many things,
from logs down a river to pollen across a garden.
Microfluidic systems, or labs-on-a-chip, use fluids to transport particles
and biological material through tiny channels. The method is often used
to count particles in order to determine a chemical's concentration.
One challenge to doing this is counteracting the Poiseuille flow effect,
which can cause particles to stick to channel walls where they will not
be counted. The same effect is present in large bodies of water. River
water, for example, flows more slowly near the shore than in the center
of the channel, making particles or biological species near the water's
edge move slower than those in the middle. Over time this effect causes
particles to spread from the middle of a channel to its sides.
Researchers at Harvard University have come up with a way to work with
the Poiseuille effect to guide particles without letting them stick to
the sides.
Current microfluidics methods counteract the Poiseuille, using one of
three methods, said Gary Zabow, a graduate student of atom-optics at Harvard
University.
One method is to make channels non-stick by spraying them with repellent.
But coating the side walls will not repel everything, and it won't stop
the spreading action.
Another method is to put two fluids in a channel, one inside the other.
But eventually, the particles of the inner fluid diffuse into the outer
fluids. Having two fluids also requires a bigger channel.
A third method is to use the electric charge of the particles. Most biological
entities, including DNA, have a natural electrical charge and so can be
moved using an electric current. Particles that do not have a natural
charge can be moved along by attaching to them beads which are affected
by an electric current.
The Harvard method uses this third method, but also works with the Poiseuille
flow effect and another innate property of fluids: the capillary force.
The capillary force determines the curvature of a fluid's surface. Water
in a thin test tube, for instance, has a downward curve, with its sides
slightly higher than the middle. Mercury, on the other hand, has an upward
curve, with the middle higher than the sides. The curvature of these liquids
with pressure. "If you flow more or less fluid at higher or lower pressure
the curvature will change," said Zabow.
By controlling both the curvature and the Poisseuille effect, the researchers
were able to guide the flow of particles within a fluid.
To do this they built a doughnut-shaped channel half filled with water
and magnetic beads, and used pressure to control the curve of the water.
The bottom half of the channel was hydrophilic, or water-attracting, and
the top half hydrophobic, or water-repelling. "The surface is pinned at
the line where the hydrophilic and hydrophobic regions meet," said Zabow.
Making the water height stationery "enables... changing pressure to translate
into a changing curvature. Without the [hydrophilic-hydrophobic] division
a changing pressure will translate instead into a changing water level,
not a changing curvature," he said.
Than they applied a magnetic force to cause the particles to concentrate
in the middle of the channel. "If you hold a speaker magnet above the
channels, the magnetic particles will cluster to the center," said Zabow.
The same is true of passing an electric charge overhead. The direction
of the curve determines where the particles concentrate. If the surface
of the liquid dips downwards, the particles collect at the sides. If the
surface bulges upwards, the particles congregate in the middle of the
channel. "The higher the arch, the more they want to go... to the middle,"
he said.
The researchers' prototype uses a permanent magnet that sits several centimeters
away from the chip and does not interfere with its working, said Zabow.
It is important that the method used to generate the electric or magnetic
field does not use power, because this would produce heat. "Having no
heating is often important because biological things don't like their
temperature to change. If your body temperature changes by one degree,
you very much know it. So if you're playing with little bio-things down
a channel, and the temperature goes up by one degree or two, they could
die or change in some way," said Zabow.
The system could be used as an accumulator or as a separator, he said.
For accumulation, the surface would curve upwards to focus the particles
in the center of the channel. Imagine that something valuable is dispersed
in the fluid, said Zabow. "You could flow a swimming pool through the
[system and] concentrate or purify the thing that was in it," because
the electrically charged particles would get sucked up to the surface,
he said.
This could be used to extract a tiny amount of DNA from a crime scene
or sense small amounts of environmental chemicals, Zabow said. "You could
stick this into the output of a factory and check out what toxic things
they're shoving out in the stream. It may be at a very low concentration
that you wouldn't be able to see normally." But with this apparatus, you
could concentrate and analyze it, he said.
As a separator, the system would have a downward curve to make particles
flow away from the center and spread, diffusing through the fluid, and
eventually collecting at the sides. Scraping them from the sides of the
tiny channels can be difficult, however, said Zabow.
There is a limit to how large the system can be because the capillary
forces are not strong enough if the channels are more than a few millimeters
wide. Smaller channels make the system more efficient. The researchers
demonstrated that the method worked in channels with diameters as large
as 4 millimeters and as small as one tenth of a millimeter, Zabow said.
The work is a clever combination of previously reported concepts, said
David Beebe, an associate professor of biomedical engineering at the University
of Wisconsin at Madison. "It [is the] first time I've seen [work that]
combines surface tension effects dominant at the microscale with electromagnetic
forces to migrate particles," he said.
While the concept is potentially useful, it may have limited application
because biological molecules, such as proteins, cells, sub-cellular components,
and DNA "tend to not like liquid-air interfaces," said Beebe. Proteins,
for example, denature, or break down, when they come in contact with air,
he said.
Another limitation is the system requires external power, said Beebe.
This means "the system won't be small and cheap, which is fine for some
applications, but not for truly portable, low cost applications."
The system is ready for practical use, said Zabow. "It's very easy to
add to an existing [microfluidics system.]" The researchers plan to further
explore different ways of achieving similar results, he said. One possibility
is looking at the effect of curvature of the electromagnetic field rather
than of the fluid, he said.
Zabow's research colleagues were Fabiano Assi, Robert Jenks, and Mara
Prentiss. They published the research in the February 25, 2002 issue of
Applied Physics Letters. The research was funded by the National Science
Foundation (NSF).
Timeline: Now
Funding: Government
TRN Categories: BioMEMS and Microfluidics
Story Type: News
Related Elements: Technical paper, "Guided Microfluidics
by Electromagnetic Capillary Focusing," Applied Physics Letters, February
25, 2002.
Advertisements:
|
April
3/10, 2002
Page
One
Electron waves compute
Software orchestrates
Web presentations
Porous glass makes
minuscule sensor
Internet map improves
models
Magnets channel biomatter
News:
Research News Roundup
Research Watch blog
Features:
View from the High Ground Q&A
How It Works
RSS Feeds:
News | Blog
| Books 
Ad links:
Buy an ad link
Advertisements:
|
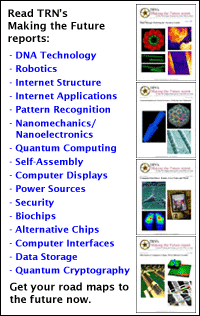
|
|
|