Biochip
sprouts DNA strands
By
Kimberly Patch,
Technology Research News
In addition to DNA's natural role of manufacturing
the proteins that drive many of life's processes, the molecule has serious
potential as a scientific tool. Researchers have found it invaluable for
sensing and identifying microbes and chemicals, and there are many possibilities
that involve using DNA to construct structures molecule by molecule.
The key to expanding DNA's sensor function is being able to quickly expose
DNA molecules that contain different sequences of nucleotides to a solution
containing substances to be tested. And the key to using DNA to build
molecular structures is finding efficient ways to position and connect
the molecules.
Researchers from the University of California at Davis and Wayne State
University have addressed both issues with a method for attaching DNA
strands to a gold surface so that the molecules stand up like a thick
forest of branchless trees.
The method also allowed the researchers to precisely position lines of
vertical DNA strands in patterns as narrow as 10 nanometers, or about
100 times the width of a hydrogen atom. "We succeeded in grafting DNA
molecules with nanometer precision at desired positions on surfaces,"
said Gang-yu Liu, an associate professor of chemistry at the University
of California at Davis. A nanometer is one millionth of a millimeter.
Because the molecules are standing up, chemicals introduced into a surrounding
solution would have access to the full strands, which stretch to heights
of around 700 nanometers. This makes the method a good candidate for making
biological microarrays that would use different types of DNA to test very
small samples of many different substances at the same time.
Such closely packed, miniature arrays could speed gene identification,
disease diagnoses, drug discovery and toxicological research, said Liu.
"Further miniaturization could provide [better] performance in a shorter
time," she said. For instance, larger portions of an organism's genome
could be incorporated into a single chip.
The ability to control exactly where DNA molecules attach also makes the
method useful for producing molecular-scale devices for applications like
quantum computing, she said. Many quantum computing schemes, which exploit
the traits of particles like atoms and electrons to store and manipulate
information, require devices small enough to direct small numbers of particles,
or even single atoms or electrons.
The researchers made their DNA patterns using chemistry and an atomic
force microscope that directly manipulates molecules using an extremely
sharp tip as narrow as one nanometer.
They prepared a surface for the DNA to attach to by making a sandwich
of mica, a thin layer of gold and a single layer of organic molecules.
They added a solution containing short, single-stranded segments of DNA
that were thiolated, meaning they contained a segment on one end capable
of adsorbing, or chemically attaching to, the gold.
When the researchers used an atomic force microscope to etch patterns
into the molecule layer, exposing the gold, strands of DNA attached to
the exposed areas. "Molecules in selected regions of the surface are shaved
away [and] the resist molecules are removed, [DNA molecules] immediately
adsorb onto these newly exposed areas following the scanning track of
the AFM tip," said Liu.
Using the same microscope tip, but less pressure, the researchers were
able to confirm that the DNA had attached by sensing the height of the
molecules on the surface. "DNA molecules are densely packed in, and adopt
a standing-up configuration," said Liu.
The ability to construct patterns of DNA and examine the results as the
patterns are being constructed is unusual, said Liu. It makes the process
easier by allowing patterns to be extended without the need to change
masks or repeat the entire fabrication process, said Liu.
The DNA the researchers used, in contrast to the variety found in living
beings, was single-stranded and very short, containing between 12 and
35 of the four bases that make up the bulk of DNA. Biological DNA stored
in the nuclei of cells contains billions of bases and is wound into a
double-stranded helix; the two strands separate to access segments of
bases that act as blueprints for building proteins.
There are two challenges to using the method practically, said Liu. The
first is to stabilize the DNA nanostructures over time. This is a necessary
step in being able to produce nano-sized features without the structures
deforming, she said.
The second is to make the structures more quickly and efficiently by making
the fabrication process parallel and more automatic. The current scanning
probe lithography process is "serial... with relatively low throughput,"
she said.
The researchers are looking to eventually use the method to form complicated
two- and three-dimensional nanostructures, said Liu. At the same time,
they will determine how the DNA nanostructures react to various agents,
she said. "Our ultimate goal is to construct designed nanostructures of
DNA, and to demonstrate their unique physical and biochemical reactivities,"
she said.
The technique is potentially useful, and could allow for smaller bioarrays
that are around 10,000 times more complex than those made using conventional
processes like robotic spotting and photolithography, said Linette Demers,
a chemist at NanoInk, Inc.
In contrast to the 10-nanometer-thick lines produced by the researchers,
robotic spotting equipment cannot currently make dots smaller than several
hundred thousand nanometers, said Demers. At the same time, the most advanced
commercial photolithography processes cannot make feature smaller than
130 nanometers.
Bioarrays -- patterns of DNA spots arranged on chips -- are widely used
to study gene structure and expression in basic research areas like oncology,
toxicology, neurology and pharmacogenomics.
The technique could also be used to improve on-the-spot diagnosis, said
Demers. Even "modest improvements in understanding and implementation
of DNA patterning and readout technologies... have an impact in biomedicine,"
she said.
There is work to be done before the researchers' technique can be implemented
in widely used real-world applications, Demers added. In its present form,
nanografting is a serial technique and thus inherently very slow. "Speed,
and the ability to lay down tens of thousands of different DNA sequences
on a chip" are needed to use the technique to miniaturize genetic arrays,
she said.
However, even slow nanografting is potentially useful for niche applications
like examining the effects of nanoscale confinement of protein molecules,
investigating new readout methods for miniaturized bioanalysis devices,
and preliminary research into bioelectric circuits, she said.
It is difficult to predict when the method could be ready for use because
of the rapid pace of change in nanotechnology development, said Liu. "We
hope to have it ready in five to fifteen years," she said.
Liu's research colleagues were Christine S. Chow of Wayne State University,
and Maozi Liu and Nabil A. Amro of the University of California at Davis.
They published the research in the August 14, 2002 issue of Nano Letters.
The research was funded by the National Science Foundation (NSF) and the
University of California at Davis.
Timeline: 5-15 years
Funding: Government, University
TRN Categories: Chemistry; Nanotechnology
Story Type: News
Related Elements: Technical paper, "Production of Nano Structures
of DNA on Surfaces," Nano Letters, August 14, 2002.
Advertisements:
|
November
13/20, 2002
Page
One
Coax goes nano
Webs within Web boost
searches
Circuit gets more
power from shakes
Method measures quantum
quirk
Biochip sprouts DNA strands
News:
Research News Roundup
Research Watch blog
Features:
View from the High Ground Q&A
How It Works
RSS Feeds:
News | Blog
| Books 
Ad links:
Buy an ad link
Advertisements:
|
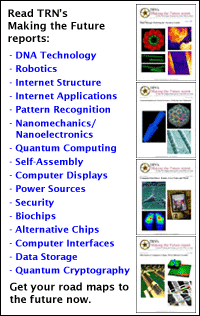
|
|
|