Altered
protein orders metal bits
By
Eric Smalley,
Technology Research News
Genetic engineering usually means experimental
drugs, altered food crops and the specter of a new race of superhumans.
Researchers from NASA, the SETI Institute and Argonne National Laboratory
have genetically modified a bacteria that lives in geothermal hot springs
in order to make a microscopic scaffolding that produces a high-tech material.
The altered bacteria gene produces a protein that automatically constructs
orderly arrays of microscopic bits of gold or zinc. The microscopic bits
of metal can serve as quantum dots, which trap one or a few electrons,
and can be used in electronics.
Nanoscale arrays of quantum dots could be used to make data storage devices
that hold enormous amounts of information; quantum computers that use
quantum dots to compute, and materials that channel light waves in optical
computers and communications devices.
The technique uses biotechnology and chemistry in place of conventional
chipmaking processes. "The concept of using self-assembling biomolecules
for materials science is not new," said Andrew McMillan, a research scientist
at NASA's Ames Research Center. What is new is "we engineered [specific
functions] into a protein that self-assembles into flat crystals," he
said.
Certain portions of the protein bind to certain metals. When the protein
tubes arrange themselves in a lattice, or crystal-like, structure, the
result is uniform placement of nanoscale bits of metal, said McMillan.
The researchers used a protein from Sulfolobus shibatae, an extremophile
organism that can grow in temperatures as hot as 85 degrees Celsius. The
bacteria's ability to withstand high temperatures means the protein, called
chaperonin, is particularly stable.
Chaperonins are essential proteins that exist in nearly all organisms,
said McMillan. Their predominant function is thought to be facilitating
protein folding inside cells, he said. Proteins fold to change shape at
the molecular level, which allows them to carry out specific life processes.
Chaperonins are made of 14, 16 or 18 protein subunits arranged in a pair
of stacked rings. "Our work takes advantage of the... characteristic barrel
shape," said McMillan. The rings are 16 to 18 nanometers high by 15 to
17 nanometers in diameter. A nanometer is about the width of 10 hydrogen
atoms.
The researchers used one of the three types of proteins that make up the
chaperonin subunits. They altered the Sulfolobus genes to produce a protein
with a slightly different structure, then inserted the altered genes into
common E. coli bacteria, which manufactured large amounts of the modified
protein. They heated the E. coli to 85 degrees Celsius to destroy the
E. coli and its own proteins, leaving behind the engineered Sulfolobus
protein.
The researchers engineered two variants of the protein. To get one variant,
they removed a portion of the gene that causes bits of protein to partially
block the openings at the ends of the chaperonin. The partially blocked
variant had an opening of 3 nanometers and the unblocked variant had an
opening of 9 nanometers.
They also mutated the genetic code of both variants to produce cysteine
residues on the chaperonins' openings. Cysteine, an amino acid, acts like
glue to bind a bit of gold or zinc to each opening like sticking a ball
to the end of a tube.
The modified chaperonins did not lose their inherent stability or ability
to self-assemble, said McMillan. "We were able to alter the protein without
destroying its ability to form interesting structures," he said.
The researchers crystallized the altered chaperonins into flat, hexagonally
packed templates, said McMillan. These chaperonin crystals arranged 5-nanometer
quantum dots into arrays when the researchers used the variant with the
3-nanometer opening, while the the 9-nanometer chaperonin arranged 10-nanometer
quantum dots.
The researchers' work expands the rapidly growing field of using biomolecules
as nanoscale scaffolding to organize inorganic nanocrystals, said Shuguang
Zhang, a principal research scientist and associate director of the Center
for Biomedical Engineering at the Massachusetts Institute of Technology.
The researchers used a well-understood protein complex that can assemble
itself into useful structures, he said.
Proteins are particularly useful because researchers can modify their
structures in precise locations without significantly altering their folding
behavior, said Zhang. "This tailor-made approach will have tremendous
impact on the growth of nanotechnology and nanobiotechnology," he said.
"However, much effort is still needed to reduce the high cost of production
and [improve the] stability of proteins in their complexes," said Zhang.
"I think the basic idea is neat, but the end result was not all that impressive,"
said Gerard Wong, an assistant professor of materials science and engineering
at the University of Illinois at Urbana Champaign. "You have to compare
what they wind up making with what people have already accomplished using
block copolymer lithography and other competing technologies," he said.
Block copolymer lithography uses ultraviolet light to alter the structure
of a mix of two polymers so that they form thin plastic films with closely
packed arrays of nanoscale holes. The films can serve as templates for
making data storage media or optical materials.
Protein-guided nanostructures could be used in practical applications
in two to five years, said Jonathan D. Trent, a research scientist at
NASA Ames Research Center. Sony Corporation and Matsushita Electric Industrial
Co., Ltd. are pursuing protein-based techniques for manufacturing memory,
he noted.
The researchers' next step is to see what other kinds of particles they
can array using their protein scaffolding, said McMillan. "We hope to
build on this model of self-assembling and see what we can push it to
do," he said.
One challenge to using the technique for practical applications is determining
whether and how to remove the protein templates, which could contaminate
devices, after the arrays are formed, said McMillan.
McMillan and Trent's research colleagues were Chad D. Paavola of NASA's
Ames Research Center, Jeanie Howard and Suzanne L. Chan of the SETI Institute,
and Nestor J. Zaluzec of Argonne National Laboratory. They published the
research in the November 25, 2002 issue of the journal Nature Materials.
The research was funded by NASA, the U.S. Department of Energy (DoE) and
the Defense Advanced Research Projects Agency (DARPA).
Timeline: 2-5 years
Funding: Government
TRN Categories: Biotechnology, Materials Science and Engineering,
Nanotechnology
Story Type: News
Related Elements: Technical paper, "Ordered Nanoparticle
Arrays Formed on Engineered Chaperonin Protein Templates," Nature Materials,
November 25, 2002
Advertisements:
|
January
1/8, 2003
Page
One
Interface gets the point
Altered protein
orders metal bits
Hubs increase Net risk
Electron pairs power
quantum plan
Aligned fields
could speed storage
News:
Research News Roundup
Research Watch blog
Features:
View from the High Ground Q&A
How It Works
RSS Feeds:
News | Blog
| Books 
Ad links:
Buy an ad link
Advertisements:
|
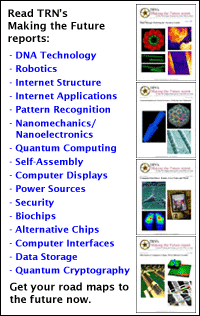
|
|
|