Quantum computing catches the
bus
By
Eric Smalley,
Technology Research News
Before researchers can build large-scale
quantum computers, they must work out ways to shunt information between
computer components.
Quantum computers use traits of particles like atoms and electrons
to compute, and are theoretically many orders of magnitude faster than
today's computers in solving very large problems, including the number-factoring
problems whose complexity underpins today's computer security software.
The challenges in building practical quantum computers include
preserving the fragile quantum states of particles that represent the
1s and 0s of digital information and controlling the delicate interactions
between particles that the computers tap to process information.
National Institute of Standards and Technology (NIST) researchers
have tapped an aspect of classical computers and a pair of weird particle
traits to allow distant particles, or qubits, to communicate as though
they were in contact.
In today's computers, memory and processor chips pass data back
and forth through a central communications bus. In contrast, many proposed
quantum architectures shunt information between particles, or qubits by
passing the information through every qubit in between, bucket-brigade
fashion. Transferring information this way is slow and error prone.
The researchers' scheme uses empty qubits as a communications
bus that allows distant memory bits to exchange information directly.
"Memory qubits... do not need to be swapped throughout the computer,"
said Gavin Brennan, a physicist at NIST. This helps cut down on errors,
which increase with each information transfer, he said.
The particles that make up qubits have states that can represent
the 1s and 0s of computing. Electrons, for example, have two different
spins, much like a top that can spin clockwise or counterclockwise.
Quantum computers have the potential to be phenomenally fast due
to a couple of weird traits of particles. When particles are isolated
from their environments, they enter the quantum state of superposition,
and are in some mix of all possible states.
And when two or more particles in superposition come into contact,
they can become entangled, meaning one or more of their properties are
linked. The link remains even if the particles are separated, and if one
particle interacts with its environment and is knocked out of superposition
into a definite state, the other particle also leaves superposition and
assumes the same state at the same instant regardless of the distance
between them.
The key to quantum computers' potential is that qubits in superposition
can represent every possible answer to a problem at the same time, allowing
the computer to check all the answers with one set of operations. Quantum
computers containing thousands of qubits would be able to solve problems
that have so many possibilities it would take today's computers longer
than the life of the universe to check them all serially.
In the nearest-neighbor quantum computer architectures that could
be improved by the NIST scheme, neighboring qubits become entangled in
order to pass along information during logic operations. The bus qubits
in the researchers' scheme don't carry information directly, but become
entangled to form a communications channel.
When distant memory qubits A and B need to interact, "one creates
a chain of... entangled pairs of bus qubits between A and B using nearest-neighbor
interactions," Brennan said. In a second step, entanglement swapping,
the ends of the chain are entangled with each other, Brennan said. Qubits
A and B can communicate by having qubit A interact with the bus qubit
at one end of the chain and B interact with the bus qubit at the other
end of the chain. "The effect is the same as if A and B interacted directly,"
he said.
The nearest-neighbor interactions needed to form a chain with
entangled ends are easier to carry out and less error prone than the interactions
needed to pass information from one qubit to the next.
A communications bus "will certainly be necessary for large quantum
computers that use nearest-neighbor interaction," said David Kielpinski,
a postdoctoral fellow at the Massachusetts Institute of Technology. The
NIST method "is innovative and plausible, drawing on interesting recent
results in quantum communication," he said.
Setting up quantum communications links between distant parts
of a quantum computer to allow widely separated qubits to talk to each
other reduces the computing time considerably, said Kielpinski. At the
same time, however, "it takes some extra work to set up a good communications
link, so more resources and time are needed than for proposals that don't
have the nearest-neighbor limitation in the first place," he said.
Proposals to build quantum computers from quantum dots, semiconductor
impurities and optical lattices all use nearest-neighbor architectures.
Quantum dots are specks of semiconductor that trap individual or small
numbers of electrons. Semiconductor impurities are individual atoms embedded
in semiconductor material. Optical lattices are three-dimensional arrays
of laser beams that trap individual atoms.
Other quantum computing architectures, including designs that
use ions trapped in magnetic fields or electric current flowing through
superconductor loops, do not link qubits via nearest-neighbor connections,
and instead include a type of fixed, common bus. Though these schemes
avoid the overhead of the researchers' communications channels, the common
buses could be a source of crosstalk that could degrade computations,
said Brennan.
There are several challenges to building large-scale quantum computers
that need to be overcome before it will be possible to implement the researchers'
communications scheme, including building hardware with precisely positioned
and readily controllable qubits, and coming up with reliable basic logic
gates.
One well-known proposal by Bruce Kane of the University of Maryland
calls for using the properties of phosphorus atoms embedded in silicon.
"Designing a regular array... is a technical challenge," said Brennan.
It would be a similar challenge to isolate the single atoms trapped in
optical lattices, he said.
Researchers agree that it will be a long time before quantum computers
become practical. "We expect a modest sized -- about 50 qubits -- quantum
architecture [in] the next 10 to 20 years," said Brennon. A 50-qubit computer
could provide simulations of complex systems better than classical computers,
he said.
Brennan's research colleagues were Daegene Song and Carl J. Williams.
The researchers posted the work on the arXiv physics archive in January,
2003. The research was funded by the National Security Agency (NSA) and
the Defense Advanced Research Projects Agency (DARPA).
Timeline: 10-20 years
Funding: Government
TRN Categories: Quantum Computing and Communications
Story Type: News
Related Elements: Technical paper, "A Quantum Computer Architecture
Using Nonlocal Interactions," posted to the arXiv physics archive, January
6, 2003.
Advertisements:
|
February 26/March 5, 2003
Page
One
Handhelds gain space
Ceramic yields under
pressure
Virtual DNA replicates
Quantum computing
catches the bus
News briefs:
Stamp
bangs out plastic circuits
DNA
forms nano piston
Alloy
could lower fuel-cell cost
Lasers
drive tiny toolset
Film
promises massive storage
Word
bursts reveal hot topics
News:
Research News Roundup
Research Watch blog
Features:
View from the High Ground Q&A
How It Works
RSS Feeds:
News | Blog
| Books 
Ad links:
Buy an ad link
Advertisements:
|
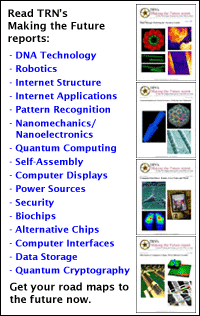
|
|
|