Logic clicks with ratchet
By
Eric Smalley,
Technology Research News
A long-shot but intriguing candidate to
replace today's computer chip architecture is cellular automata.
Cellular automata are large arrays of simple, identical components,
or cells. Each cell can be switched between states that can represent
the 1s and 0s of computing, and chain reactions along lines of cells can
transmit this information within cellular automata to allow them to carry
out the basic logic operations of computing.
Logic circuits made from the devices would be extremely fast and
use very little power.
One challenge to making such computer circuits is finding ways
to coax the cells to change from a 1 to a 0, and vice versa. Researchers
at Los Alamos National Laboratory have come up with a ratchet-based scheme
for forming logic units from cellular automata.
The design represents bits of information as the positions of
microscopic, tornado-like electrical currents, or vortices, in superconducting
material. Superconductors have little resistance to electricity at very
low temperatures and so allow electrons to pass through them without losing
energy. Superconductor vortices form anywhere a magnetic field penetrates
a superconductor.
The vortices ordinarily move around, but can be confined by adding
defects, or wells, to the crystal structure of the superconductor. "You
can think of the vortices as point-like objects that repel each other,
but are trapped in the wells," said Hastings. The researchers' design
calls for rows of vertical wells. A single vortex can be trapped at the
top or bottom of the well but not in between.
A well could represent 1 when its vortex is at the top and 0 when
it is at the bottom.
In order to transmit data and perform logic operations within
the device, a vortex at the top of one well would have to be able to force
the vortex in an adjacent well to the bottom to initiate a chain reaction
that would flip the positions of the vortices in a row of wells.
But simply changing the position of the vortex in the first well
is not enough to switch the second well because the second vortex faces
as much resistance from the vortex in third well as it does from the newly
repositioned first vortex, said Hastings.
The researchers' ratchet system consists of three types of vertical
wells -- one narrow followed by two wide. One type of wide well is designed
to make its vortex favor the left side, and the other on the right. The
ratchet action begins with vortices at the top of the narrow well, the
bottom of the middle well, and the top of the last well.
Moving the first vortex down causes the second vortex to move
up because it is closer horizontally to the first than to the third, and
so feels a stronger repulsion from the first than from the third, said
Hastings. Applying an electric current to the ratchet forces the right
hand vortex to shift to the left, which in turn pushes the vortex down,
completing the flip.
The current causes all of the middle vortices in an array of ratchet
devices to shift at once. "Using this approach, [you] can propagate information
along the row of wells," said Hastings. "With a refinement of this ratchet,
you can build gates and fanouts to perform logic operations by acting
on several rows of vortices," he said.
Although the researchers designed the device to be built from
superconductors, the scheme could be used with other cellular automata
hardware, said Matthew Hastings, a researcher at Los Alamos National Laboratory.
The ratchet mechanism makes it possible to build very small logic gates,
he added.
The superconductor-based device, however, will work only at very
low temperatures, said Hastings. "Since the system must work below the
superconducting transition temperature, we will not have computers based
on this device sitting on our desktops." It could be used in supercomputing
applications, he said.
The researchers are working on fabricating arrays of wells, and
are also looking into building a cellular automata ratchet mechanism out
of quantum dots and out of Josephson junction vortices. A quantum dot
is a tiny bit of semiconductor material that traps a single electron,
and a Josephson junction is a superconducting circuit that has a microscopic
break that causes electrons flowing through the circuit to behave like
a single giant subatomic particle.
Unresolved issues include determining how precisely the wells
need to be made, and how to input data to and read results from the device,
said Hastings.
The method could be used practically in about a decade, according
to Hastings. The timeframe would "depend greatly and what is found when
the experiments are done [and] how rapidly other competing forms of logic
advance," he said.
Hastings research colleagues were Cynthia J. Olson Reichart and
Charles Reichart. The work appeared in the June 20, 2003 issue of Physical
Review Letters. The research was funded by Los Alamos National Laboratory.
Timeline: 10 years
Funding: Government
TRN Categories: Integrated Circuits; Logic; Physics
Story Type: News
Related Elements: Technical paper, "Ratchet Cellular Automata,"
Physical Review Letters, June 20, 2003; Web movies at www.t12.lanl.gov/home/olson/VCA.html.
Advertisements:
|
July 16/23, 2003
Page
One
Sensors guard privacy
Cheaper optics-chip
link on tap
Logic clicks with
ratchet
Electricity shapes
nano plastic
News briefs:
Experience handed
across Net
3D display goes vertical
Gel yields nanotube
plastic
Nano toolbox
gains carbon cones
Jolts mix micro fluids
Jet-laser tandem
prints gold
News:
Research News Roundup
Research Watch blog
Features:
View from the High Ground Q&A
How It Works
RSS Feeds:
News | Blog
| Books 
Ad links:
Buy an ad link
Advertisements:
|
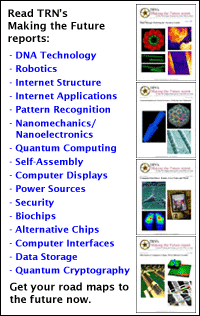
|
|
|