Bank
transfer demos quantum crypto
By
Eric Smalley,
Technology Research News
A
wire transfer from Vienna City Hall via a cable running through the sewer
system to a bank a few blocks away has marked the first real-world use of
cryptography that takes advantage of the weird quantum phenomenon of entanglement.
Particles like atoms, electrons and photons can all be entangled,
and in the entangled state, particle properties like spin and polarization
remain linked regardless of the distance between the particles.
Although commercial quantum cryptography systems use the properties
of individual particles to encode information, they do not use entangled
particles. And although the first laboratory demonstration of entanglement-based
quantum cryptography occurred in 1998, the Vienna wire transfer is the first
public demonstration that entangled particles can survive the rigors of
practical application.
On April 21, 2004, researchers from the University of Vienna in
Austria, ARC Seibersdorf Research GmbH in Austria, the University of Munich
in Germany, and the Austrian Academy of Sciences transferred a secret encryption
key using the perfectly secure entangled-state quantum cryptography demonstration
system connecting Vienna City Hall and Bank Austria Creditanstalt.
The entangled-state system guaranteed that each bit of information
was represented by just one photon, making it impossible for an eavesdropper
to peek at the information without being detected. The entangled-state system
is also perfectly random, making it impossible for an eavesdropper to gain
information about the code by examining the equipment used.
A photon's electric field can be polarized, or oriented, in one
direction within two sets of opposite directions -- horizontal-vertical
and the two diagonals. Pairs of opposite directions can represent binary
numbers. For example, horizontal can represent 1 and vertical 0.
Particle properties like polarization can become entangled when
two or more particles come into contact with each other or simultaneously
interact with a third entity like another particle or a laser beam. The
entangled particles exist in the quantum state of superposition -- a mix
of all possible states. The researchers' photons were not polarized in any
one direction until they were observed.
Quantum cryptography systems exchange encryption keys encoded in
particles like photons, and use the keys to protect messages. The systems
are theoretically perfectly secure because according to the laws of physics
an eavesdropper cannot observe a string of individual particles without
giving away his presence.
Existing systems use weak laser pulses that contain one or several
photons, said Andreas Poppe, a researcher at the University of Vienna. These
extra photons could potentially be siphoned off using a beam-splitter. With
only one photon per bit, an eavesdropper would have to replace the photons
he intercepted, but these photons would not be entangled with the sender's
half of each photon pair. This would be easy to detect because it would
lead to a high error rate -- many of the polarizations would not match up.
The entangled-state system is also perfectly random by the laws
of nature, making it impossible for an eavesdropper to gain information
about the code by examining the equipment used. The weak-laser systems require
the photon polarizations that represent the encryption key to be set before
they are transmitted. The systems use random number generators to determine
polarizations of strings of photons. Random number generators are never
truly random, however, and in theory, an eavesdropper could observe a pattern
that could help crack the code.
Using the demo system in Vienna City Hall, University of Vienna
physics professor Anton Zeilinger and Vienna mayor Michael Häupl pressed
a button to create a string of entangled photons and transmit one photon
from each entangled pair to a sending station and the other to a receiving
station, according to Poppe.
To build a key, the sender and receiver measure their photons and
tell each other which of the two pairs of polarizations they looked for,
but not the results of the measurements. When one of a pair of entangled
photons is measured, it becomes polarized and at the same instant the other
photon becomes polarized in the opposite direction. The polarizations are
random and known only to the sender and receiver. A string of entangled
photons produces a secret random number that can be used to scramble a message
at the sending end and unscramble it at the receiving end.
In order to be perfectly secure, a signal must use a single photon
to represent each bit of information. If information is encoded in multiple
photons, an eavesdropper can siphon off some of the photons to observe the
information and leave the sender and receiver none the wiser. If he looks
at information encoded in a single photon, however, the observation destroys
the photon. Even if he replaces the photon, the sender and receiver could
see that the replacement photon was not entangled with its erstwhile mate,
revealing the presence of an eavesdropper.
The system transmitted the secret key bits at 80 bits per second.
Once the Vienna banking demonstration encryption key was established,
Zeilinger and Häupl pressed a transmit button to send the ordinary encrypted
data that made up the wire transfer.
In the researchers' experiment, the encrypted data was sent over
the same fiber as the secret key for convenience. The data was transmitted
using the Internet's TCP/IP protocol and in principle could be sent via
any Internet connection, said Poppe.
The system could eventually be used to guarantee secure communications
between parties within the same city. With the development of quantum repeaters,
the system could be extended to cover long distances. Quantum repeaters
use entanglement to refresh fading quantum signals. Ordinary telecommunications
repeaters make copies of light pulses, but copying destroys quantum information.
The researchers' system used entangled photons whose wavelength
was 810 nanometers. Their next step is to make the system work with 1,550-nanometer
light, which will travel greater distances. Photons at that wavelength are
more difficult to work with because they are hard to detect, said Poppe.
The 1,550-nanometer wavelength is one of two standard telecommunications
wavelengths.
The method could be used in practical networks in four years, said
Poppe.
Poppe and Zeilinger's research colleagues were Alessandro Fedrizzi,
Rupert Ursin and Hannes Böhm of the University of Vienna, Thomas Lorünser,
Oliver Maurhardt, Momtchil Peev and Martin Suda of ARC Seibersdorf Research
GmbH, Christian Kurtsiefer and Harald Weinfurter of the University of Munich,
and Thomas Jennewein of the Austrian Academy of Sciences.
The work appeared in the August 9, 2004 issue of Optics Express.
The research was funded by the Austrian Science Fund (FWF), the European
Commission, the Austrian Ministry of Transportation Innovation and Technology
(FIT), the City of Vienna, and the Bank Austria Creditanstalt.
Timeline: 4 years
Funding: Government; Corporate
TRN Categories: Quantum Computing and Communications; Cryptography
and Security; Physics
Story Type: News
Related Elements: Technical paper, "Practical Quantum Key
Distribution with Polarization Entangled Photons," Optics Express, August
9, 2004
Advertisements:
|
September
22/29, 2004
Page
One
Fuel cell converts
waste to power
Bank transfer demos
quantum crypto
Agent model yields leadership
Flexible sensors
make robot skin
Briefs:
Microscope
etches ultrathin lines
Nanowire makes
standup transistor
Plastics ease
nanotube circuits
Virus forms nano template
Photo molecules
flip current
Nanotubes
on cloth fire electrons
News:
Research News Roundup
Research Watch blog
Features:
View from the High Ground Q&A
How It Works
RSS Feeds:
News | Blog
| Books 
Ad links:
Buy an ad link
Advertisements:
|
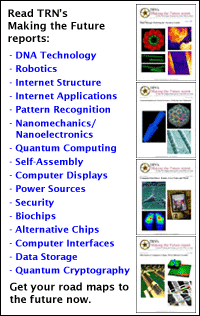
|
|
|