DNA machines take a walk
By
Eric Smalley and Kimberly Patch,
Technology Research News
Researchers
working to form nanoscale machines and materials are increasingly tapping
nature's building blocks. Two particularly helpful molecules are DNA, which
encodes instructions for making the proteins that carry out life's processes,
and the motor protein kinesin, which is part of the a cell's transportation
system.
DNA molecules contain strings of four types of bases -- adenine,
cytosine, guanine and thymine -- attached to a sugar-phosphate backbone.
Single strands self-assemble into structures like life's familiar double
helix when their bases match up. Researchers can manipulate artificial strands
of DNA by controlling these connections.
Kinesin molecules have a pair of short extensions, or legs, at one
end and a tail at the other end. The legs attach to a microtubule protein,
and step to move the kinesin bipedal molecule forward along the microtubule
molecule. The tail grabs cell structures like vesicles and mitochondria
to transport the structures over a cell's extensive network of microtubules.
Several research teams have built DNA walkers, inspired in part
by kinesin, that move along DNA tracks.
Researchers from Duke University and the University of Oxford in
England have devised a a series of DNA stations that pass a DNA fragment
from one to the next. The walker works autonomously, using enzymes present
in the environment to initiate each step of the process.
Researchers at the California Institute of Technology have built
a bipedal DNA walker that improves the gate of a walker originally designed
by researchers at New York University from shuffling, with one leg always
in back of the other, to leg-over-leg walking.
Both types of walkers could eventually be used to construct nanoscale
devices, synthesize and deliver drugs, and carry out DNA computation. "Eventually,
it may be possible to program synthetic motors that can haul diverse molecules
along intricate paths for use in nanoscale factories or molecular medicine,"
said Niles Pierce, an assistant professor of applied and computational mathematics
and bioengineering at Caltech.
The Caltech prototype is designed to mimic kinesin, and consists
of a DNA track with four anchorage sites and a bipedal DNA walker. The anchorage
sites are single strands of DNA set five nanometers apart along the double
helix track, and the walker is a double helix structure with strands separated
at one end.
The walker's loose strands form a pair of 23-base legs, and the
four anchorage sites contain different sequences of 20 bases.
To set up the process, the researchers add an attachment strand
that joins at one end with the first anchorage strand and at the other end
with one leg. A short segment in the middle of the attachment strand that
does not match up with the leg or anchorage strands provides flexibility.
To make the device take a step, the researchers add a second attachment
strand that moves the second leg by fixing it the second anchorage strand.
To make it take another step, the researchers free the first leg with a
detachment strand that removes the first attachment strand, then swing the
leg forward and bind it to the third anchorage with a third attachment strand.
In this way, the walker progresses one leg in front of the other along the
track.
The prototype falls short of kinesin in several regards, according
to Pierce. Kinesin runs autonomously whereas the researchers' DNA walker
requires DNA fuel strands -- the attachment and detachment strands -- to
be administered at each step, he said. Kinesin also moves at about 100 steps
per second while the researchers' device takes around two steps per hour,
he said.
The Duke University walker consists of a double-stranded DNA track
with three DNA anchorage site segments attached to the track by single-strand
hinge segments of DNA. The anchorage DNA is double-stranded, but one strand
shorter, leaving a three-base extension at the free end. The walker is a
six-base single strand.
The walker begins attached to the first anchorage. The three bases
at the free end of the walker attach to the end of the second anchorage,
causing the first and second anchorages to swing on their hinges toward
each other. An enzyme frees the walker from the first anchorage and alters
the anchorage to keep the walker from stepping backwards. The free end of
the walker then attaches to the third anchorage. A second enzyme cuts the
walker free from the second anchorage to complete the move to the third
anchorage site.
Unlike the bipedal DNA walkers, which require DNA strands to be
added at each step of the process, the Duke device operates continuously
because none of its components interfere with each other and so can all
be present in the environment. "Our walker operates in an autonomous fashion
while previous constructions by other groups require... the adding and removal
of fuel DNA strands to drive the walker," said Hao Yan, a Duke University
researcher who is now an assistant professor of chemistry and biochemistry
at Arizona State University.
The DNA walker could eventually be used to carry out computations
and to precisely transport nanoparticles of material, according to Yan.
The walker can be programmed in several ways. "For example, we can encode
information in [the] walker fragments as well as in the track so that while
performing motion, the walker simultaneously carries out computation," said
Yan.
The walker could also be programmed to transport tiny bits of material,
said Yan. "If integrated with a well-defined large-scale nanostructure such
as two-dimensional DNA nano-grids, the walker might be able to precisely
transport a nanoparticle from one location to another location on the nanostructure...
in a programmable and autonomous fashion," he said.
The DNA walkers advance DNA nanotechnology by anchoring controlled,
progressive motion to a structure. This is a key step toward harnessing
the work of DNA machines. "Ultimately, our objective in pursuing rational
DNA design is to develop a molecular compiler that takes as input a conceptual
design for a device and produces as output a list of DNA sequences that
can be expected to assemble into the desired system," said Caltech's Pierce.
It is likely to take 10 years or more to design and build practical
molecular motors from scratch, said Pierce. The Duke DNA walker could be
used practically in five years, said Yan.
Yan's research colleagues were Peng Yin, Xiaoju G. Daniell, Andrew
J. Turberfield and John H. Reif. Their work appeared in the Sept. 27, 2004
issue of Angewandte Chemie International Edition. The research was
funded by the National Science Foundation (NSF).
Pierce's colleague was Jong-Shik Shin. Their work appeared in the
July 14, 2004 issue of Nano Letters, and was funded by the Defense
Advanced Research Projects Agency (DARPA), the Charles Lee Powell Foundation,
the Ralph M. Parsons Foundation, and Caltech.
Timeline: 5 years; 10 years
Funding: Government
TRN Categories: DNA Technology; Nanotechnology
Story Type: News
Related Elements: Technical paper, "A Unidirectional DNA Walker
That Moves Autonomously along a Track," Angewandte Chemie International
Edition, September 27, 2004; technical paper, "A Synthetic DNA Walker for
Molecular Transport," Nano Letters, July 14, 2004
Advertisements:
|
November 3/10, 2004
Page
One
Ultrathin carbon
speeds circuits
DNA machines take a walk
DNA in nanotubes
sorts molecules
Single field shapes
quantum bits
Briefs:
Nanotubes
lengthen to centimeters
Coated nanotubes
record light
Photonic crystal
lasers juiced
Lasers move droplets
Molecules form
nano containers
Square rings
promise reliable MRAM
News:
Research News Roundup
Research Watch blog
Features:
View from the High Ground Q&A
How It Works
RSS Feeds:
News | Blog
| Books 
Ad links:
Buy an ad link
Advertisements:
|
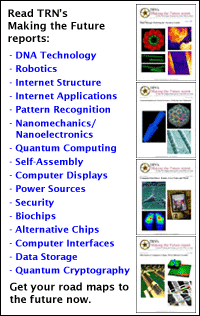
|
|
|