Laser
boosts liquid computer
By
Eric Smalley,
Technology Research News
The most advanced experimental quantum
computers use the same technology medical magnetic resonance images use
to make images of our insides.
MRIs make images of soft tissue by aligning the body's hydrogen atoms
with a continuous magnetic field, and then using pulses from other magnets
to knock the hydrogen atoms out of alignment in the areas being imaged.
After each pulse, the atoms realign with the continuous magnetic field
and in the process they emit radio waves whose frequencies are specific
to different types of tissue.
Nuclear magnetic resonance (NMR) quantum
computers make qubits,
which represent the ones and zeros of computing, by flipping the orientations
of atoms within the molecules of a liquid. However, it's extraordinarily
difficult to read the spin flips of more than a half a dozen of these
qubits, or quantum bits, at a time.
Although a growing number of researchers are calling NMR quantum computing
a dead end, some who are pressing ahead with the technology have moved
it a small step forward.
The seemingly insurmountable problem with NMR quantum computers is that
as they gain more qubits the signals from the qubits get weaker and are
eventually drowned out by the random noise of the system. The largest
NMR quantum computer built to date consists of seven qubits. They're not
likely to get much bigger using current designs, and it will probably
take thousands of qubits to make a practical quantum computer.
A team of researchers at Stanford University and IBM Research, however,
has found a way to strengthen the signals from a two-qubit NMR quantum
computer.
Nuclear magnetic resonance devices manipulate atoms magnetically in order
to detect them. When some types of atoms are placed in a strong external
magnetic field, their nuclei emit radio signals. The nucleus of an atom
behaves like a tiny magnet, a property called spin. In order for nuclear
magnetic resonance to work, enough atoms have to be polarized, or have
their spins aligned either with or opposite an external magnetic field,
that the collective signal cuts through the noise produced by randomly
oriented atoms.
The signal decreases as the number of qubits increases in NMR quantum
computers, said Anne S. Verhulst, a researcher at Stanford University.
But because the NMR signals are "proportional to the polarization of the
nuclear spins, our technique increases the signals. Hence we can allow
more qubits before the signals disappear in the noise," she said.
To do this, the researchers mixed rubidium vapor with xenon gas and aimed
a laser into the mixture. The laser light was circularly polarized, so
that its electric field rotated. The laser polarized, or aligned the electrons
of the rubidium atoms, which in turn polarized the xenon. The researchers
then separated out the xenon, froze it into a liquid and mixed it with
liquid chloroform. The xenon polarized some of the carbon and hydrogen
atoms in the chloroform, and those atoms served as the stronger qubits
in the researchers' quantum computer.
Boosting the polarization this way increased the strength of the spin
signals by a factor of 10, said Verhulst. In order to use the scheme on
a practical level, the researchers will have to increase the strength
of the signals by 1,000 times and show that it works on computers made
of more than two qubits, she said.
Despite the success of the initial results, the odds are still heavily
against NMR yielding practical quantum computers. "Even though NMR quantum
computers are the only ones existing so far, the problems related to the
scaling issue are really huge. If one wants to make it work with liquids,
then either some very special molecules or NMR technique or some additional
source of high polarization has to be found, or a combination of all these
things. And all of those are not straightforward to discover," she said.
The research doesn't have to lead to practical quantum computers to be
useful, said John M. Myers, a project scientist at Harvard University,
who helped build a 5-qubit NMR quantum computer in 1999.
"What is worthwhile about this research is the advance of molecular control,"
said Myers. "The techniques of NMR quantum computing can help to determine
structures of large molecules, such as proteins. That is something special
about NMR, in contrast to other quantum information processing schemes,"
he said.
It will take at least 15 to 20 years before practical quantum computers
can be built, said Verhulst. "A lot of the efforts involve… nanofabrication,
building extremely sensitive probes, trying to manipulate single electrons.
And all of those are interesting for technological evolution as a whole,"
she said.
Verhulst's research colleagues were Oskar Liivak and Mark H. Sherwood
of IBM Research, Hans-Martin Vieth of the Free University of Berlin, and
Isaac L. Chuang now at the Massachusetts Institute of Technology. They
published the research in the October 8, 2001 issue of the journal Applied
Physics Letters. The research was funded by the Defense Advanced Research
Projects Agency (DARPA).
Timeline: 15-20 years
Funding: Government
TRN Categories: Quantum Computing
Story Type: News
Related Elements: Technical paper, "Non-Thermal Nuclear
Magnetic Resonance Quantum Computing Using Hyperpolarized Xenon," Applied
Physics Letters, October 8, 2001
Advertisements:
|
October
24, 2001
Page
One
DNA could crack code
Transistor sports
molecule-thin layer
Molecule connects contacts
PC immortalizes ancient
temple
Laser boosts liquid computer
News:
Research News Roundup
Research Watch blog
Features:
View from the High Ground Q&A
How It Works
RSS Feeds:
News | Blog
| Books 
Ad links:
Buy an ad link
Advertisements:
|
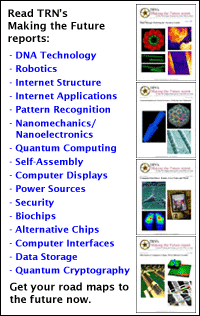
|
|
|