Prefab key to molecular memory
By
Kimberly Patch,
Technology Research News
It is one thing to put together a device
that works. It is another matter entirely to reliably manufacture thousands
of them.
This is one of the challenges facing researchers working on nanodevices
-- electrical and mechanical components whose sizes range down to the
atomic scale. Such devices promise to use molecules as super-fast computer
circuits, store fantastic amounts of information in a minuscule area and
sense minute amounts of chemicals and biological materials.
Researchers from the University of Southern California, NASA Ames
Research Center and Rice University have brought these possibilities a
step closer with a way to gently add electrical contacts to a sheet of
molecules to produce molecular memory.
The method increases the yield of viable memory by 30-fold, up
from only 1 or 2 percent, said James Tour, a professor of chemistry, computer
science, mechanical engineering and materials science at Rice University.
The method is "a solution to the long-standing electric contact
issue in making molecular devices," said his colleague Chongwu Zhou, an
assistant professor of electrical engineering at the University of Southern
California.
Molecular memory devices consist of a layer of molecules sandwiched
between metal electrodes. Electric current flows from one electrode to
the other through the molecule-thick layer. A high voltage jolt flips
the resistance of the molecules, making it easier or more difficult for
the current to pass through. A low voltage can measure the resistance
of the molecules, and the two resistance levels can represent the 1s and
0s of computer information.
The devices have the potential to store binary information in
small numbers of molecules. In addition, smaller electronics would be
faster, simply because the currents of electrons that carry signals don't
have as far to travel. "Molecular electronics in general has potential
to produce ultra-dense and high-performance integrated electronic systems,"
said Zhou.
To make the molecular memory, the researchers used beams of electrons
to form four parallel gold electrodes 150 nanometers wide and spaced 200
nanometers apart. A row while 1,500 hydrogen atoms spans 150 nanometers,
which is about one seventh the diameter of an E. coli bacterium.
The researchers chemically coaxed a single layer of molecules
to assemble on top of the electrodes, and scratched the molecules off
one electrode using an atomic force microscope. They then put pre-made
palladium nanowires across the electrodes.
The researchers formed the 10- to 15-nanometer wide nanowires
ahead of time by coating carbon nanotubes with palladium. Nanotubes are
rolled-up sheets of carbon atoms, and can be smaller than a single nanometer,
or the span of 10 hydrogen atoms.
The nanowires formed a direct contact with the electrode where
the monolayer was scratched off, and formed junctions with the molecules
at the intersections of the other three electrodes.
Forming the top contacts ahead of time makes it possible to gently
apply top electrical contacts to a small assembly of molecule-coated electrodes,
said Zhou. The method "eliminates long-standing problems like thermal
damage and poor yield related to defects in the monolayer," he said. Top
contacts are usually added using metal evaporation techniques that subject
the device to high temperatures.
The researchers used the method to test several types of molecules
and show that they can be used to store information.
They are still working out how the molecules actually switch resistance
states. "There are two possibilities," said Zhou. Either a physical twist
of the molecules, termed conformation transition, or a chemical change
-- a reduction oxidation, or redox reaction. "Our work shed some light
on this [but] it is still an open issue," he said. "My best guess is that
the memory effects are caused by an interplay of redox and conformation
transition," he said.
The researchers are working on constructing prototype molecular
memory chips that will hold one kilobit, or 1,000 bits, said Zhou. Today's
state-of-the-art computer memory chips hold a gigabit, or one billion
bits of information.
In the near-term the higher yield method should allow researchers
to make prototype devices more easily. "This can inspire more work toward
molecular memory chips... though substantial challenge remains ahead,"
said Zhou.
It will be more than five years and maybe more than 10 before
the method can be used to build practical molecular memory chips and circuits,
said Zhou.
Tour and Zhou's research colleagues were Chao Li, Daihua Zhang,
Xiaolei Liu, Song Han and Tao Tang at the University of Southern California;
Wendy Fan, Jessica Koehne, Jie Han and Meyya Meyyappan from NASA Ames
Research Center; and Adam M. Rawlett and David W. Price from Rice University.
The work appeared in the January 27, 2003 issue of Applied Physics Letters.
The research was funded by the National Science Foundation (NSF).
Timeline: > five years
Funding: Government
TRN Categories: Data Storage Technology; Nanotechnology
Story Type: News
Related Elements: Technical paper, "Fabrication Approach
for Molecular Memory Arrays," Applied Physics Letters, January 27, 2003
Advertisements:
|
June 18/25, 2003
Page
One
Chip sorts colors
Software referees group
calls
Prefab key to molecular
memory
Wires make wireless
strain gauge
News briefs:
See-through circuits
closer
Protein traps nanoparticles
Nods drive audio
interface
Nano rapid prototyping
advances
Practical nanotube
fiber near
Nanotube transistors
make memory
News:
Research News Roundup
Research Watch blog
Features:
View from the High Ground Q&A
How It Works
RSS Feeds:
News | Blog
| Books 
Ad links:
Buy an ad link
Advertisements:
|
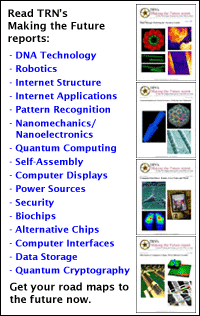
|
|
|